Exposing the potential of sugar chains for the diagnosis and treatment of disease
17 June 2011
Research into cellular sugar chains is revealing a series of new properties and functions with potential application in the diagnosis and treatment of disease
Naoyuki Taniguchi
Team Leader, Disease Glycomics
Team Group Director, Systems Glycobiology Research Group
RIKEN Advanced Science Institute
Protruding from the surface of cells in the body like whiskers are sugar chains, a biological structure often bound to lipids and proteins embedded in the cell membrane. Recent studies have shown that sugar chains exhibit a broad range of functions, including signal transduction between cells and across the cell membrane, as well as functional regulation of immunity and hormones. “From among the diverse functions of sugar chains, we focus on their association with disease,” says Naoyuki Taniguchi, group director of the Systems Glycobiology Research Group at the RIKEN Advanced Science Institute and a world-renowned researcher in sugar chains. “The ultimate goal of our research is to clarify the mechanisms of the onset of disease in terms of sugar chains, and to diagnose and treat disease using those mechanisms.” Taniguchi’s research is probing the frontiers of sugar chain science for the diagnosis and treatment of disease.
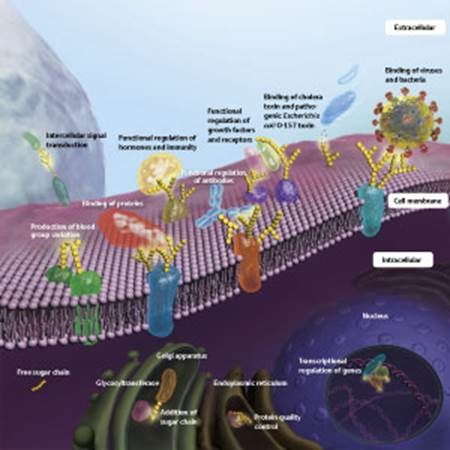
Figure 1: Various functions of sugar chains
“The human body consists of about 60 trillion cells, and nearly all of them have sugar chains protruding from their surfaces, rather like whiskers,” says Taniguchi. The sugar chain is literally a chain of monosaccharide sugars. “Traditionally, it has been thought that the primary functions of sugar chains are to store energy and build biological structures. However, it has recently been shown that most sugar chains occur in the form of glycoproteins or glycolipids, in which they are bound to lipids or proteins embedded in the cell membrane.” About 50% of proteins bear sugar chains, and those sugar chains have diverse functions (Fig. 1).
The sugar chains bound to proteins or lipids comprise a sequence of several and occasionally up to several thousands of units of various kinds of sugars, which join together and branch in complex ways. The sugar chains exposed to cell surfaces are structurally distinct from each other depending on the type of cell, and even within the same kind of cell their structure changes under different conditions.
“The sugar chain is like the face of the cell,” says Taniguchi. “When we communicate with other people, we look at their face to identify them. Likewise, cells, as well as proteins and many other biological molecules, recognize sugar chains exposed to cell surfaces and bind to them to achieve mutual communication. Viruses, bacteria and pathogenic toxins also recognize sugar chains, bind to them, and invade cells. When cells become cancerous, the sugar chains change and adopt a structure specific to cancer cells. Hence, the sugar chain comes to be the ‘face’ of the cancer cell.”
Although sugar chains are involved in all biological phenomena, including development, differentiation and immunity, they have only been studied actively in the last 15 years or so. “The sugar chain is also called ‘the third chain of life’. However, it is much more difficult to study than the first and second chains of life—DNA and proteins,” says Taniguchi. DNA comprises a chain of bases, and protein comprises a chain of amino acids. There are four different bases constituting the DNA, and 20 amino acids make up protein. There are about ten monosaccharides that constitute sugar chains in humans, which link to each other in complicated ways and sometimes branch. As a result, there is no practical apparatus for amplifying or synthesizing sugar chains in the same way as exists for DNA and proteins.
Nevertheless, our understanding of sugar chains has grown thanks to the discovery of genes for glycosyltransferases, which elongate sugar chains by attaching sugars one by one, as well as improvements in mass analyzers and other instruments for examining the structures of sugar chains. “It has been found that sugar chains are important for biological activities and are closely associated with disease. We are working to understand the mechanisms of the onset of disease by studying sugar chains with the aim of linking them to the diagnosis and treatment of disease with sugar chains.”
Sugar chains as biomarkers
Blood tests now routinely screen for γ-GTP (gamma glutamyl transpeptidase), PSA (prostate specific antigen) and other parameters. These are called biomarkers, and they are used for the early detection of diseases such as impaired liver function or liver cancer (γ-GTP) and prostate cancer (PSA). Biomarkers are molecules that change in abundance and composition with the onset and progression of disease, and can serve as effective diagnostic indicators.
“Most currently available biomarkers are glycoproteins or glycolipids. For example, an antibody that binds specifically to the protein moiety of a glycoprotein is created, and the protein is quantified. However, this does not provide an accurate diagnosis because there is no difference between the proteins of glycoproteins produced by normal cells and those produced by cancer cells,” says Taniguchi. In fact, many people have elevated PSA levels but do not have prostate cancer, whereas others have normal PSA levels even though they have prostate cancer.
Figure 2: Change in γ-GTP sugar chain.
The γ-GTP sugar chain in normal cells is bifurcated. If a cell becomes cancerous, the glycosyltransferase GnT-III acts to bind a sugar called N-acetylglucosamine to the branching base to form a bisect structure.
More than 20 years ago, Taniguchi proposed for the first time in the world that sugar chains, rather than the proteins in glycoproteins, could be used as biomarkers. “The structures of sugar chains are known to change due to the attachment or removal of their sugars upon onset of disease,” he explains.
In 1983, Taniguchi demonstrated that the γ-GTP sugar chain in normal cells is bifurcated, and that the γ-GTP sugar chain of cancer cells assumes a ‘bisect’ structure with one molecule of the N-acetylglucosamine sugar bound to the base of the branch (Fig. 2). He later discovered the gene for the glycosyltransferase GnT-III, which catalyzes the formation of the structure. “By utilizing structural changes in sugar chains, it is possible to examine the cancerization of cells earlier and more accurately. When cancer starts, up to several molecules of fucose sugar attach to the structures of alpha-fetoprotein, which is a biomarker for liver cancer, and haptoglobin, which is a biomarker for pancreatic cancer. A technology for detecting alpha-fetoprotein with one fucose attached to it is already available for practical use, and we are now working to develop biomarkers for a variety of cancers based on structural changes in sugar chains to detect various cancer cells.”
A role in chronic obstructive pulmonary disease
Another research theme for the Disease Glycomics Team is to clarify the role of sugar chains in chronic obstructive pulmonary disease (COPD), such as lung edema and bronchitis, and to develop therapeutic drugs for the disease. In Japan, it is estimated that 200,000 patients are being treated for COPD, but the potential patient population may be up to 5.3 million. Primary causal factors are smoking, including passive smoking, and air pollution. COPD is becoming more prevalent worldwide, and the World Health Organization predicts that the disease will become the fourth leading cause of death by 2020.
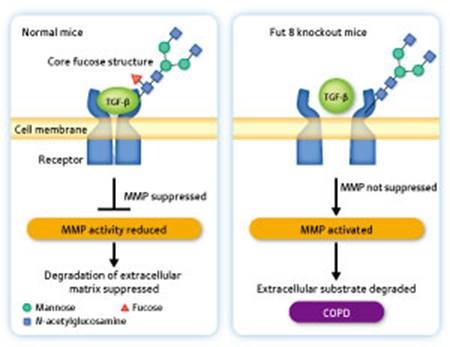
Figure 3: Mechanism of onset of chronic obstructive pulmonary disease (COPD).
Left: In the pulmonary cells of a normal mouse, a core fucose structure is present in the sugar chain of the TGF-β receptor. TGF-β binds to the receptor, producing a signal for suppressing the expression of matrix metalloprotease (MMP). Right: In the pulmonary cells of a Fut 8 knockout mouse, no core fucose structure is present in the sugar chain of the TGF-β receptor. Therefore, TGF-β is unable to bind to the receptor and no signal is produced for suppressing the expression of MMP. As a result, the alveolar wall collapses and alveoli swelling, resulting in the onset of COPD.
The Disease Glycomics Team did not target COPD at the beginning, but took up the case when Taniguchi discovered Fut 8, a core fucose structure formed when glycosyltransferase attaches fucose to the base of a sugar chain. Taniguchi and his colleagues generated a knockout mouse lacking the gene for Fut 8 to examine the function of the core fucose structure. “Seventy percent of our Fut 8 knockout mice died within three days of birth. The mice that survived suffered symptoms of COPD, such as alveolar swelling, around three weeks after birth. Human COPD patients were found to have lower blood Fut 8 concentrations in accordance with the severity of their condition. This finding strongly suggests that Fut 8 is involved in the onset of COPD, and it motivated us to investigate the relationship between sugar chains and the onset of COPD.”
The swelling of alveoli—the tiny air sacs in the lungs—occurs due to excess activation of matrix metalloprotease (MMP), a protein-degrading enzyme, which causes the alveolar wall to collapse. In normal mice, the TGF-β molecule binds to its receptor in the cell membrane to generate a signal for suppressing the expression of MMP, thus preventing the collapse of the alveolar wall (Fig. 3). “When we examined the sugar chain attached to the TGF-β receptor, we found that the core fucose structure was present in normal mice but absent in Fut 8 knockout mice. Because the Fut 8 knockout mice lack the core fucose structure, TGF-β cannot bind to the receptor in their body. As a result, the signal for suppressing the expression of MMP is not functioning, so the alveolar wall is destroyed by the activated MMP. This proved to be the mechanism of the onset of COPD.”
No radical therapies are available for COPD, which is currently treated merely by symptomatic approaches, such as with steroids or bronchodilators. Patients with COPD can suffer rapid, sometimes fatal, exacerbation of their symptoms upon viral or bacterial infection. “Since we demonstrated the association of sugar chains in the onset of COPD, we have been working on creating a new therapeutic drug that targets sugar chains,” says Taniguchi. Although sugar-chain-targeting therapeutics may sound strange, in fact influenza remedies target sugar chains, while many other currently available therapeutic drugs target proteins.
Enhancing antibody pharmaceutical activity
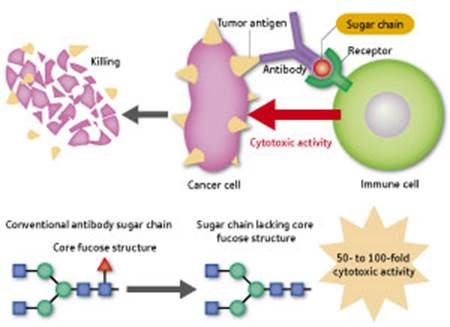
Figure 4: Action mechanism of antibody pharmaceuticals.
While binding to cancer cell-specific antigens, antibodies also bind to immune cells such as macrophages and natural killer cells. Activated immune cells attack and kill cancer cells (upper panel). The activity for cancer cell attack is increased 50- to 100-fold by removing one fucose from the sugar chain on the antibody to make the antibody free of the core fucose structure (lower panel).
“Research into Fut 8, which we discovered, is also expected to lead to improvements in antibody pharmaceuticals,” says Taniguchi. Many cancer therapeutics, including Herceptin for breast cancer, are categorized as antibody pharmaceuticals—antibodies that recognizes a specific antigen on cancer cell surfaces and when administered to a patient binds not only to cancer cells, but also to immune cells, such as macrophages and ‘natural killer’ cells. These immune cells are thus activated by the antibody and attack and kill the cancer cells (Fig. 4). “Ordinary antibody pharmaceuticals have an attached sugar chain with a core fucose structure produced by Fut 8. Recently, two pharmaceutical companies, one in Japan and the other in the US, independently demonstrated that merely removing the fucose from the sugar chain enhances its activity against cancer cells by 50 to 100 times. This approach is applicable to antibody pharmaceuticals for a broad range of cancers, so there are great expectations for it.”
Sugar chains are also closely related to cancer metastases. The glycosyltransferase GnT-V, which was discovered around the same time independently by Taniguchi and a US research group, attaches N-acetylglucosamine to the end of a sugar chain to bifurcate it. Cancer cells having a sugar chain with this structure are highly metastatic, whereas those having a sugar chain with a bisect structure produced by the glycosyltransferase GnT-III are non-metastatic. “It has been confirmed that cancer metastases decrease with the transfer of GnT-III to cancer cells. We are now working on this glycosyltransferase in the hope of developing new cancer therapeutics.”
An era of systems glycobiology
As sugar chains are involved in all biological phenomena and diseases, the scope of research at the Disease Glycomics Team is broad. In addition to the work described above, diverse studies are being conducted under the leadership of Shinobu Kitazume, deputy head of the laboratory, including the development of an early diagnostic for Alzheimer’s disease using sugar chains attached to a β-amyloid precursor protein, and the development of a new type of neovascularization inhibitor that targets sugar chains.
Taniguchi’s Systems Glycobiology Research Group is comprised of three teams: Disease Glycomics (headed by Naoyuki Taniguchi), Glycometabolome (headed by Tadashi Suzuki) and Structural Glycobiology (headed by Yoshiki Yamaguchi). “I think we’re the first in the world to announce that we’re a laboratory for systems glycobiology,” says Taniguchi. “Traditionally in glycoprotein research, researchers have specialized in sugar chains, proteins or compounds, and there has been almost no overlap. However, the functions of glycoproteins cannot be fully understood unless not only sugar chains, but also proteins and compounds, are investigated from a broad perspective. I have therefore proposed the concept of systems glycobiology.”
The Systems Glycobiology Research Group belongs to the Chemical Biology Department (directed by Hiroyuki Osada) in the RIKEN Advanced Science Institute. Chemical biology is the discipline for investigating the mechanisms behind biological phenomena using compounds. In RIKEN, a compound bank, the Natural Products Depository (NPDepo), systematically collects, preserves and supplies natural compounds of microbial origin. “We have begun screening the collection of the NPDepo in search of compounds that act on sugar chains to suppress the onset of Alzheimer’s disease, COPD and the growth or metastasis of cancer cells.”
A joint project undertaken by the Chemical Biology Department and the Max Planck Institute in Germany is also about to start. “Researchers at Max Planck became aware of the research into chemical biology and sugar chains being done at RIKEN and asked if they could work with us. Japan has a long history of sugar chain research and has been leading the world with outstanding achievements, including the discovery of 60% of the genes for glycosyltransferases. The Max Planck Institute also has a world-renowned sugar chain researcher and runs a large compound bank. By working with them, we want to drive systems glycobiology more powerfully.”
Sugar chain imaging is the ultimate goal of Taniguchi’s work on sugar chains. “I want to watch movie images of sugar chains to find out which sugar chains are present in what amounts at which locations in the body in real time. Researchers into sugar chains have long dreamed of this. We are determined to achieve it within a few years, to help understand the functions of sugar chains and link the findings to the diagnosis and treatment of disease.”
About the Researcher
Naoyuki Taniguchi
Naoyuki Taniguchi was born in Tokyo, Japan, in 1942 and grew up in Sapporo. He graduated from the Faculty of Medicine at Hokkaido University and obtained his MD degree in 1967 and then PhD degree in 1972 from the same university. He became assistant professor of the Department of Preventive Medicine at Hokkaido University, and later visiting associate professor at the Connell University Medical School in New York, USA, in 1976. After returning to Japan, he became associate professor of the Cancer Institute of Hokkaido University Medical School, where he started his career in glycobiology. In 1986, he became professor and chair of the Department of Biochemistry at Osaka University Medical School. In 2006, after retirement from the medical school, he became an endowed chair professor of Osaka University. He launched the Systems Glycobiology Research Group at the RIKEN Advanced Science Institute in 2007, and has been leading his group as group director. He is focusing on the structure and function of glycans, especially the role of N-linked glycoproteins in relation to the mechanism of disease, biomarker discovery and therapeutics.